1. Introduction
2. Experimental
2.1. Chemicals
2.2. Precipitation procedure and analytical methods
3. Results and Discussion
3.1. Effect of reaction temperature
3.2. Effect of volume ratio of solvent to Li(I) solution
3.3. Effect of the molar ratio of carbonate to Li(I) in the solution containing acetone or ethanol
3.4. Effect of pH adjustment during precipitation by (NH4)2CO3
3.5 Effect of reaction time
4. Conclusions
1. Introduction
Lithium is an essential metal with various applications, mainly in batteries (35%) and ceramics and glass (32%), due to its outstanding properties such as electrochemical activity, lowest reduction potential, and highest specific heat1). Lithium-ion batteries (LIBs) with excellent properties such as high energy density, long life with long charge-discharge cycles, and extensive operating temperature range are essential energy sources used in advanced portable electronic devices and electric vehicles2). LIBs account for about 37% of the world's rechargeable battery market, and the number of these products is constantly expanding at a fast rate every day1). Along with the development of LIBs production technology, recycling of spent LIBs is also a matter of concern for environmental and economic purposes. Spent LIBs are important secondary resources for metals such as lithium, nickel, cobalt, and manganese3). Intensive research has been done on metal recovery from spent LIBs, but most works focus on the recovery of Ni and Co rather than Li. Namely, the lithium recycling rate from LIBs is only <1%1). Current industrial processes that recycle LIBs do not recover enough lithium to meet the demand from LIBs manufacturers for electric cars4). Therefore, Li recovery is an urgent and essential issue for the sustainable development of the supply of LIBs for modern industry.
A combined process of pyrometallurgy and hydrometallurgy for spent LIBs can facilitate the selective recovery of valuable metals5), and the smelting reduction of spent LIBs leads to the formation of alloys, Al-Li-containing slags, and Li-containing dust6). In the previous study, dust containing Li was selectively dissolved under optimal conditions: weight ratio of Li dust to solid NaOH of 1.00:0.17, 50 g/L pulp density, 22°C, 120 min. Table 1 shows the composition of the leaching solution obtained at this condition7). Among several kinds of Li compounds industrially produced such as Li2CO3, LiOH, LiCl, LiBr, and C4H9Li, Li2CO3 is an essential component in all LIBs1,4). Therefore, it is necessary to separate, purify and recover lithium from the leaching solution in the form of Li2CO3 precipitate. In this work, two precipitants for Li(I) in an aqueous solution were used, namely Na2CO3 and (NH4)2CO3. The addition of non-aqueous solvents such as acetone, ethanol, and ammonia to aqueous solutions reduces the dielectric constant of the solution, which affects the solubility of compounds. Therefore, the effect of several variables such as the molar ratio of carbonate to Li(I), solution pH, the nature of non-aqueous solvents, reaction temperature, and time were investigated. Moreover, the precipitation behavior of Li(I) was compared between Na2CO3 and (NH4)2CO3.
Table 1.
The concentration of metal ions in the leaching solution by distilled water from the dust of the smelting reduction of spent LIBs7)
Ion | Li(I) | Na(I) |
Concentration, mg/L | 3514.5 | 5606.1 |
2. Experimental
2.1. Chemicals
The concentration of metal ions in the leaching solution by distilled water from the dust produced from the smelting reduction of spent LIBs is shown in Table 17). The solutions containing Li(I) and Na(I) were synthesized by dissolving a certain amount of chloride salts of the respective metals, such as lithium chloride (LiCl, Sigma-Aldrich, >99.0%) and sodium chloride (NaCl, Showa Chemical Co., >99.5%) in doubly distilled water to the desired concentrations. The pH of the solution was adjusted by HCl (Daejung Chemical & Metals Co., >35.0%) and NaOH (Duksan Co., >99.0%). The carbonate salts, including ammonium carbonate ((NH4)2CO3, Daejung Chemical & Metals Co., >30.0%) and sodium carbonate (Na2CO3, Daejung Chemical & Metals Co., >99.0%) were used as the precipitants. Non-aqueous solvents such as ammonia solution (NH3, Junsei Chemical Co., >30.0%), acetone ((CH3)2CO, Daejung Chemical & Metals Co., >95.0%), and ethanol (C2H5OH, Daejung Chemical & Metals Co., >95.0%) were used without further purification.
2.2. Precipitation procedure and analytical methods
The precipitation experiments were performed in a 250 mL Erlenmeyer flask and the reaction conditions such as stirring speed, time, and temperature were controlled by a magnetic stirrer (WiseStir MSH-20D, Daihan Scientific Co., Seoul, Korea). The pH of the solution was determined by an Orion Star thermal scientific pH meter (model A221, USA). The precipitates were separated from the filtrate using filter paper (ADVANTEC No. 2, 110 mm, Toyo Roshi Kaisha, Ltd.). The concentration of Li(I) in the filtrate was measured by ICP-OES (Inductively coupled plasma-optical emission spectrometry, Spectro Arcos). The precipitation percentage was calculated as where ms and mf represent the mass of Li(I) in the leaching solution and the filtrate.
3. Results and Discussion
3.1. Effect of reaction temperature
The concentration of Li(I) and Na(I) in the synthetic solution for Li(I) precipitation experiments was 3514.5 mg/L and 5606.1 mg/L, respectively and the solution pH was 10.4. As a precipitant, Na2CO3 and (NH4)2CO3 were added to the solution. Table 2 shows that the solubility of Li2CO3 in water decreases with increasing temperature8). Therefore, the experiments were at two temperatures of 60 and 90°C by varying the molar ratio of carbonate to Li(I) from 0.5 to 1.5. In these experiments, the stirring speed was fixed at 400 rpm and the reaction time was 60 min. The precipitation reaction of Li2CO3 is represented as Eq. (1) and the variation of the solubility product (Ksp) with temperature is also shown in Table 28,9).
Table 2.
Solubility and solubility product constant (Ksp) of lithium carbonate in water at various temperatures8,9)
Temperatures | 0°C | 10°C | 20°C | 30°C | 40°C | 60°C | 80°C | 100°C |
Solubility | 1.54 | 1.43 | 1.33 | 1.26 | 1.17 | 1.01 | 0.85 | 0.72 |
Ksp | - | - | - | 4.278×10-4 | - | 2.031×10-4 | 8.864×10-5 | - |
Fig. 1 shows the variation in the precipitation percentage of Li(I) with the molar ratio of carbonate to Li(I) using Na2CO3 and (NH4)2CO3 at two reaction temperatures of 60 and 90°C. In the case of using Na2CO3, the carbonate participating in the precipitation with lithium is provided by the dissociation according to the equation
In an aqueous solution, carbonate ions can react with hydrogen ions. However, the protonation reaction of carbonate would not occur in an alkaline solution because the concentration of hydrogen ions is too small. Therefore, the dissociated carbonate can participate in the precipitation reaction. With the increase in the molar ratio of carbonate to Li(I), the Li2CO3 precipitation percentage did not change significantly, around 70% at 90°C and around 40% at 60°C. Our data indicate that consideration of the solubility of Li2CO3 is important in obtaining the precipitates by reaction with carbonate. Since the solubility of Li2CO3 decreases with increasing temperature, the amount of precipitate obtained at 90°C was more than 60°C in both cases of Na2CO3 and (NH4)2CO3 under the same reaction conditions (see Fig. 1). When (NH4)2CO3 was used as a precipitant, the carbonate participating in the precipitation with Li(I) is provided by the dissociation according to the equation
Unlike sodium ions, ammonium ions are weak acids and can dissociate into ammonia and hydrogen ions. Since the basicity of carbonate is stronger than that of ammonia, the dissociated carbonate can be protonated by water, which can be expressed as8)
The concentration of carbonate ions that can participate in precipitation reaction would be lower in the case of (NH4)2CO3 compared to Na2CO3. Therefore, the precipitation percentage of lithium carbonate from sodium carbonate was higher than from ammonium carbonate under the same experimental conditions.
At 90°C, in the case of ammonium carbonate, the precipitation percentage of Li(I) increased from 50% to 57%, as the molar ratio of the carbonate to Li(I) increased from 0.5 to 0.7 and was constant at about 57% with the further increase of the ratio to 1.5. This can be explained by the solubility of lithium carbonate in water at various temperatures as shown in Table 28,9). In addition, the production of NH3 in the solution with pH 10.4 according to Eq. (4) would increase the solubility of Li2CO3 in the mixture of NH3 and solutions10). Fig. 1 indicates that it is difficult to completely precipitate Li(I) from the solution by varying the reaction temperature or molar ratio of carbonate to Li(I).
3.2. Effect of volume ratio of solvent to Li(I) solution
The solubility of the lithium carbonate decreases with the decrease of the dielectric constant of the solution at constant temperature11). Table 3 shows the dielectric constants of the liquids decrease in the order of water, ethanol, acetone, and ammonia solution12,13). To increase the Li(I) recovery by Li2CO3 precipitation, solvents such as acetone, ethanol, and ammonia were added to the solution containing Li(I) and Na(I) to reduce the dielectric constant of the solution.
Table 3.
Components | Dielectric constant (25°C) |
Water | 79.99 ± 0.04 |
Ethanol | 25.02 ± 0.02 |
Acetone | 21.30 ± 0.02 |
Ammonia | 16.90 ± 0.04 |
The reaction was carried out at the molar ratio of carbonate to Li(I) of 0.5 at room temperature, 400 rpm for 120 min. The volume ratio of solvents added to the solution was varied from 60% to 150% (v/v). Fig. 2 shows that the Li(I) precipitation percentage increased with the increasing volume ratio of solvents with all three solvents. Higher precipitation percentage of Li2CO3 was obtained from Na2CO3 than from (NH4)2CO3 under the same reaction conditions. When ammonia solution was added, the Li(I) precipitation percentage was below 10%. This shows that the addition of an ammonia solution increases the solubility of Li2CO3 although the dielectric constant of the ammonia solution is the lowest (see Table 3). The data from Fig. 2 shows the recovery rate of Li(I) from the solution containing acetone is higher than in ethanol because the solubility of Li2CO3 is reduced in mixtures having lower dielectric constants. However, the difference in the precipitation percentage of Li(I) was not significant due to the small difference in the dielectric constants of acetone and ethanol (see Table 3). When Na2CO3 was added as a precipitant, the precipitation percentage of Li(I) increased slightly from 90 to 95% for acetone and from 86 to 93% for ethanol as the solvent volume was increased. The precipitation percentage of Li2CO3 increased significantly from 19 to 70% for acetone and 16 to 64% for ethanol when (NH4)2CO3 was used as the volume ratio of solvents increased. In doing experiments using acetone with ammonium carbonate, it is remarkable that acetone can react with NH3 in solution to form 2-aminopropan-2-ol (2HN-C(CH3)2-OH) according to the following equation14).
However, it takes some reaction time for Eq. (7) to occur14). The formation of 2-aminopropan-2-ol was observed from the filtrate. Namely, the color of the filtrate became reddish-brown with the progress of time. More experiments are needed to investigate the effect of 2-aminopropan-2-ol on precipitation. In the scope of this study, care should be taken to filter the precipitate out of the solution immediately so as not to affect the purity of Li2CO3. From the obtained results, a volume ratio of 120% acetone or ethanol was selected as the optimal solution to recover Li(I).
3.3. Effect of the molar ratio of carbonate to Li(I) in the solution containing acetone or ethanol
Sodium carbonate and ammonium carbonate were added to a Li(I) solution containing acetone or ethanol in a 120% volume fraction to form Li2CO3 precipitate. Experiments were carried out at room temperature, 400 rpm for 120 min with a molar ratio of carbonate to Li(I) ranging from 0.5 to 1.5. In the case of sodium carbonate, the Li(I) precipitation rate did not change significantly around 94% with acetone and 93% with ethanol as the molar ratio of carbonate to Li(I) increased (see Fig. 3). The hydrolysis of NH4+ and CO32− makes the concentration of carbonate supplied from ammonium carbonate smaller than from sodium carbonate. Therefore, the Li(I) precipitation percentage by ammonium carbonate was lower than that of sodium carbonate. Specifically, the Li(I) precipitation percentage increased from 53 to 59% with ethanol and from 58 to 63% with acetone when the molar ratio of carbonate to Li(I) from ammonium carbonate ratio increased from 0.5 to 1.5 (see Fig. 3). The pH data in the filtrate after removing the Li2CO3 precipitate is shown in Table 4. The pH of the solution after the reaction did not change significantly in the case of Na2CO3 with the increase in the molar ratio of carbonate to Li(I) and thus did not affect the Li2CO3 precipitation percentage. In the case of (NH4)2CO3, due to the hydrolysis of NH4+ and CO32-, the effective concentration of CO32- which can react with Li(I) decreased, and thus the pH of the filtrate decreased. This agrees well with the data shown in Table 4. Therefore, optimal pH adjustment can greatly enhance the precipitation of Li(I) from solutions containing acetone or ethanol by (NH4)2CO3.
Table 4.
Final pH of the filtrate after the precipitation Li(I)
3.4. Effect of pH adjustment during precipitation by (NH4)2CO3
To increase the precipitation of Li(I) by ammonium carbonate, 2.0 M NaOH solution was used to adjust the pH during precipitation. The reactions were performed at room temperature for 120 min at a stirring speed of 400 rpm with a molar ratio of carbonate to Li(I) of 0.7. Fig. 4 shows that the precipitation percentage of Li(I) increased significantly when the pH of the solution increased from 9 to 12. The Li(I) recovery efficiency was about 85% when the pH of the solution was controlled at 12 for ethanol or acetone. Although it is possible to increase the precipitation percentage of Li(I) by adjusting pH to a higher value, this does not provide economic value and thus the optimal pH adjustment can be considered to be 12.
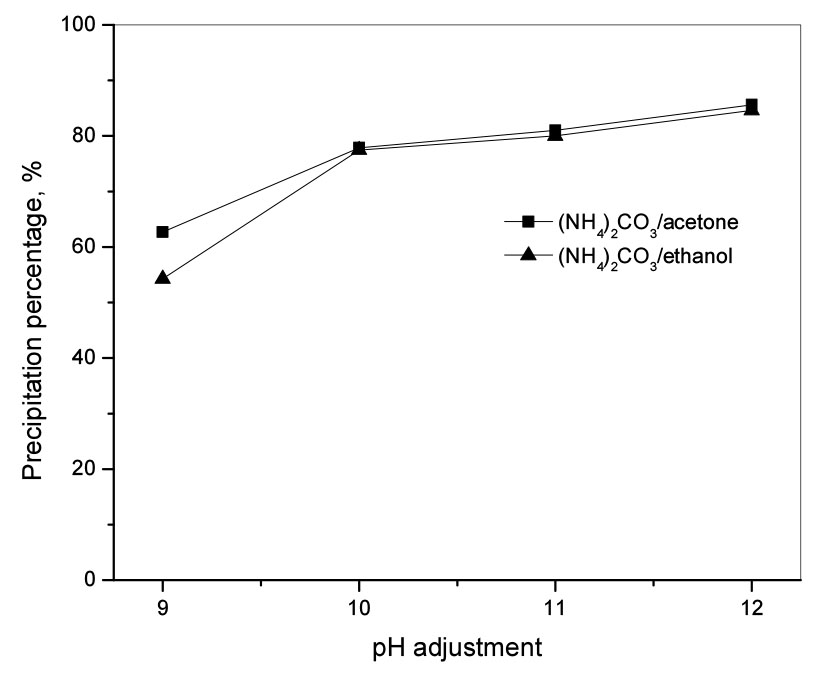
Fig. 4.
The effect of pH on the precipitation of Li(I) from the solutions containing acetone and ethanol. Operation conditions: molar ratio of (NH4)2CO3 to Li(I) = 0.7, initial pH = 10.4, volume ratio of solvent (acetone or ethanol) = 120% (v/v), T = 25°C, stirring speed = 400 rpm, and time = 120 min.
3.5 Effect of reaction time
The experiments were performed at room temperature and 400 rpm with a molar ratio of carbonate to Li(I) of 0.7 by varying reaction times from 15 min to 150 min. A volume fraction of 120% of acetone and ethanol was added to the solution containing Li(I) and the pH was adjusted to 12 during the reaction. Fig. 5 shows that the reaction time did not significantly affect the Li(I) precipitation percentage in both cases of acetone and ethanol. The precipitation percentages of Li(I) by sodium carbonate from ethanol and acetone were 93% and 94% after 15 min of reaction and were stable for up to 150 min. In the case of ammonium carbonate, the precipitation percentage reached a maximum after 30 min about 85% for both acetone and ethanol.
4. Conclusions
In order to recover Li(I) carbonate from the dust which is produced from the smelting reduction of spent LIBs, precipitation experiments were done with synthetic leaching solution of Li dust. The concentration of Li(I) and Na(I) in the synthetic solution was 3514.5 mg/L and 5606.1 mg/L and the solution pH was 10.4. Na2CO3 and (NH4)2CO3 were added to the solution as precipitants and the effect of several variables was investigated. Compared to Na2CO3, the dissolved ammonium and carbonate from (NH4)2CO3 take part in hydrolysis reactions with water, which decreases the effective concentration of carbonate. The addition of nonaqueous solvents like acetone and ethanol to solutions was effective in improving the precipitation percentage of Li(I). The highest precipitation percentage (94%) was obtained by adding acetone to the solution at room temperature when the molar ratio of Na2CO3 to Li(I) was 0.7 and the volume ratio of solvent was 120%. In the case of precipitation with (NH4)2CO3, an increase of solution pH to 12 improved the precipitation percentage of Li(I) to 86%.